Weldability of Annealed, Normalized, Quenched and Tempered Materials
Introduction
Weldability is a crucial aspect in the realm of materials engineering and fabrication, representing the ability of a material to be welded under specific conditions without compromising its structural integrity. This property is of paramount importance in various industries, influencing the selection of materials for construction, manufacturing, and other applications where welding plays a pivotal role. In this article, we delve into the nuanced world of weldability, focusing on the key considerations associated with annealed, normalized, quenched, and tempered materials.
Definition of Weldability
Weldability, in essence, refers to the ease with which a material can be joined or welded to form a robust and durable connection. It encompasses a range of factors, including the material’s chemical composition, microstructure, and the welding processes employed. The weldability of a material is not uniform across all types, and it becomes imperative to understand and evaluate this characteristic to ensure the success of welding operations.
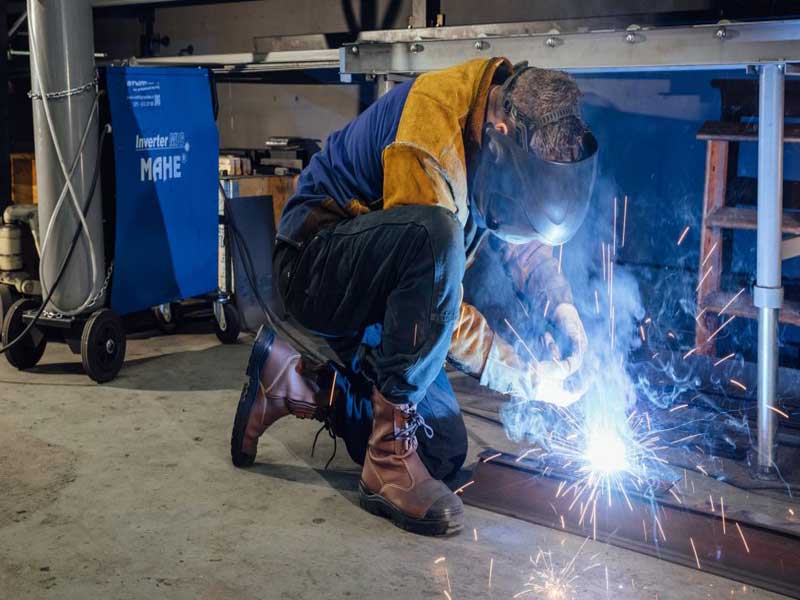
Importance of Understanding Weldability in Material Selection
The significance of comprehending weldability becomes evident in the context of material selection for various engineering applications. Different materials exhibit distinct weldability characteristics, and selecting a material with suitable welding properties is crucial to achieving desired performance, durability, and overall structural integrity. An informed understanding of weldability aids engineers and fabricators in making judicious choices during the material selection process, contributing to the overall success of a project.
Annealed Materials
Definition and Process of Annealing
Annealing is a heat treatment process employed to modify the properties of a material, typically to enhance its ductility and reduce hardness. The process involves heating the material to a specific temperature, holding it at that temperature for a set duration, and then slowly cooling it. The controlled cooling phase is crucial for achieving the desired microstructure and mechanical properties.
The primary objective of annealing is to relieve internal stresses, induce grain refinement, and create a more uniform structure within the material. This, in turn, improves the material’s machinability, formability, and overall workability.
Microstructure and Mechanical Properties of Annealed Materials
The microstructure of annealed materials is characterized by well-defined grains and a refined structure. The heat treatment process promotes the recrystallization of the material, leading to a more uniform and fine-grained structure. This, in turn, results in improved mechanical properties such as increased ductility and reduced hardness.
Annealed materials typically exhibit enhanced toughness and are less prone to cracking during subsequent manufacturing processes. The reduction in hardness also makes them more amenable to deformation and shaping, contributing to their versatility in various applications.
Factors Affecting the Weldability of Annealed Materials
Grain Size
The grain size of annealed materials plays a pivotal role in determining their weldability. Finer grain structures are generally associated with improved mechanical properties and reduced susceptibility to cracking during welding. Larger grain sizes, on the other hand, may lead to decreased toughness and increased vulnerability to weld-related defects.
Weldability considerations involve selecting appropriate heat treatment conditions that result in an optimal grain size for the specific application. Engineers and welders must balance the need for enhanced mechanical properties with the practical aspects of welding, ensuring a successful and durable joint.
Chemical Composition
The chemical composition of annealed materials significantly influences their weldability. Alloying elements, impurities, and the overall composition can affect the material’s response to welding processes. Variations in composition may introduce challenges such as increased susceptibility to cracking, changes in thermal conductivity, and altered solidification behavior during welding.
Understanding the chemical composition of the material is crucial for predicting and mitigating potential issues during welding. It guides the selection of appropriate welding techniques, filler materials, and pre/post-weld heat treatments to achieve the desired results.
Heat Input During Welding
The heat input during welding is a critical factor in the weldability of annealed materials. Excessive heat input can lead to undesired changes in microstructure, such as grain growth or the formation of undesirable phases. On the other hand, insufficient heat input may result in incomplete fusion and inadequate joint strength.
Controlling heat input through welding parameters, such as welding speed and energy input, is essential to achieve a well-balanced heat-affected zone (HAZ). This ensures that the material retains its desired properties, and the welded joint exhibits the necessary strength and integrity.
Normalized Materials
Definition and Process of Normalization
Normalization is a heat treatment process designed to enhance the mechanical properties of a material by achieving a uniform grain structure and relieving internal stresses. The process involves heating the material to a temperature above its critical transformation range, holding it at that temperature for a specified duration, and then allowing it to cool in still air. The controlled cooling results in a refined grain structure, promoting improved strength, toughness, and overall stability.
The normalization process is particularly effective for materials that have undergone prior hot-working or significant deformation, as it helps restore uniformity in grain size and mechanical properties.
Microstructure and Mechanical Properties of Normalized Materials
Normalized materials exhibit a fine and uniform grain structure, contributing to enhanced mechanical properties. The heat treatment refines the microstructure, reducing the likelihood of inhomogeneous regions within the material. This results in improved strength, hardness, and resistance to fracture.
The normalized state often brings about a balance between strength and toughness, making these materials suitable for applications where a combination of these properties is crucial. Additionally, normalized materials display improved machinability and formability, further expanding their versatility in various engineering applications.
Weldability Considerations for Normalized Materials
Grain Structure
The grain structure of normalized materials is a critical factor influencing their weldability. The fine and uniform grain size, achieved through the normalization process, contributes to improved mechanical properties and resistance to cracking during welding. It is essential to maintain this refined grain structure during welding to ensure the integrity of the joint.
Weldability considerations involve selecting appropriate welding parameters and techniques to preserve the desired grain structure. Control over heat input and cooling rates is crucial in preventing excessive grain growth or the formation of undesirable phases, which could compromise the material’s properties.
Transformation Behavior During Welding
Understanding the transformation behavior of normalized materials during welding is essential for successful welding operations. The material undergoes thermal cycles during welding, leading to changes in its microstructure. It is crucial to anticipate and manage these transformations to prevent the formation of brittle phases or the degradation of mechanical properties.
Welding techniques, such as preheating and controlled cooling, can be employed to mitigate the effects of transformation during welding. By carefully managing the thermal cycles, welders can ensure that the material retains its normalized microstructure and properties in the welded joint.
Heat-Affected Zone Effects
The heat-affected zone (HAZ) in normalized materials is susceptible to changes in microstructure and mechanical properties due to the welding process. Controlling the effects on the HAZ is crucial to maintaining the overall integrity of the welded joint. Excessive heat input can lead to undesirable transformations or grain growth in the HAZ, affecting the material’s properties.
Welding practices, including proper preheating and post-weld heat treatment, can help minimize the impact on the HAZ. By carefully managing the thermal effects on the material surrounding the weld, welders can ensure that the normalized properties extend into the HAZ, creating a welded joint with consistent and reliable characteristics.
Quenched and Tempered Materials
Definition and Process of Quenching and Tempering
Quenching and tempering is a two-step heat treatment process used to enhance the mechanical properties of materials, particularly achieving a balance between hardness and toughness. The process begins with quenching, where the material is rapidly cooled from a high temperature (usually through immersion in a quenching medium such as water or oil). This rapid cooling results in the formation of a hard and brittle phase, often martensite. Subsequently, tempering involves reheating the material to a lower temperature to achieve the desired balance of hardness and toughness.
Quenching and tempering are commonly employed to achieve specific mechanical properties in high-strength materials, making them suitable for applications where both strength and impact resistance are critical.
Microstructure and Mechanical Properties of Quenched and Tempered Materials
The microstructure of quenched and tempered materials is characterized by the presence of martensite and, after tempering, a controlled distribution of other phases. This combination imparts high strength and hardness to the material, while the tempering process provides improved toughness and reduced brittleness. The resulting mechanical properties make quenched and tempered materials well-suited for applications in demanding and high-stress environments.
Challenges and Considerations in Welding Quenched and Tempered Materials
Martensitic Transformation and its Impact on Weldability
Welding quenched and tempered materials poses challenges due to the presence of martensite, a hard and brittle phase. Martensitic transformation occurs during rapid cooling in the quenching process and can lead to the formation of cracks and other weld defects. The high hardness of martensite makes it susceptible to cracking, particularly in regions affected by the heat of welding.
Managing martensitic transformation during welding involves careful control of heat input, preheating, and post-weld heat treatment. These measures help prevent excessive hardness in the heat-affected zone (HAZ) and reduce the risk of cracking, ensuring the integrity of the welded joint.
Residual Stresses
Quenching and tempering introduce significant residual stresses into the material, and welding further contributes to the development of additional stresses. Residual stresses can lead to distortion, warping, and the initiation of cracks in the welded joint. Controlling and mitigating these stresses is crucial for the long-term performance of the welded structure.
Preheating, controlled cooling rates, and the use of appropriate welding techniques can help minimize residual stresses. Additionally, post-weld heat treatment may be employed to relieve some of the stresses and enhance the overall stability of the welded joint.
Hardenability Effects
Hardenability, the ability of a material to be hardened by heat treatment, influences the depth and distribution of hardness in quenched and tempered materials. Welding can affect the hardenability of the material, leading to variations in hardness within the welded joint. Inhomogeneous hardness distribution can compromise the mechanical properties of the joint, impacting its performance under load.
To address hardenability effects, careful consideration is given to the selection of welding parameters, including preheating temperature and heat input. By optimizing these parameters, welders can achieve a more uniform hardness distribution, ensuring that the welded joint retains the desired mechanical properties.
Welding Techniques for Different Materials
Common Welding Methods (e.g., TIG, MIG, Stick)
Welding encompasses various methods, each suited for specific applications and materials. Understanding the characteristics of common welding methods is essential for achieving successful welds.
TIG Welding (Tungsten Inert Gas)
TIG welding, also known as GTAW (Gas Tungsten Arc Welding), utilizes a non-consumable tungsten electrode to create an arc for welding. This method is versatile and suitable for a wide range of materials, including stainless steel, aluminum, and exotic alloys. TIG welding allows for precise control over the heat input and produces high-quality, clean welds, making it ideal for applications where aesthetic appearance is crucial.
MIG Welding (Metal Inert Gas)
MIG welding, or GMAW (Gas Metal Arc Welding), employs a continuously fed wire electrode and a shielding gas to protect the weld from atmospheric contamination. MIG welding is known for its efficiency and is commonly used for welding steel, aluminum, and stainless steel. It provides high deposition rates, making it suitable for industrial applications where productivity is a key consideration.
Stick Welding (Shielded Metal Arc Welding)
Stick welding, or SMAW, involves using a consumable electrode coated with a flux to create an arc for welding. This method is versatile and can be applied to a wide range of materials, including carbon steel, stainless steel, and cast iron. Stick welding is well-suited for outdoor or field welding due to its portability and the ability to work in adverse conditions.
Preheating and Post-Weld Heat Treatment
Preheating and post-weld heat treatment are crucial steps in welding, particularly for materials with specific heat treatment requirements.
Preheating
Preheating involves heating the base material to a specified temperature before welding. This helps reduce thermal gradients, minimize the risk of cracking, and promote uniform microstructural changes during welding. Preheating is especially important for materials with high hardenability or when dealing with thick sections. The temperature and duration of preheating depend on the material type and thickness.
Post-Weld Heat Treatment (PWHT)
Post-weld heat treatment is applied after welding to relieve residual stresses and optimize the material’s properties. This process typically involves heating the welded joint to a specific temperature and holding it for a predetermined time before controlled cooling. PWHT is essential for materials like quenched and tempered steels, where it helps prevent cracking and enhances the overall toughness of the joint.
Selection of Filler Materials for Each Type of Material
Choosing the right filler material is critical for achieving strong and durable welds, as it directly influences the chemical and mechanical properties of the weld joint.
Matching Filler Materials
For optimal results, the filler material should match the base material as closely as possible. This ensures compatibility in terms of composition, mechanical properties, and weldability. Matching filler materials help maintain the integrity of the joint and contribute to the overall performance of the welded structure.
Dissimilar Metal Welding
In cases where welding dissimilar materials is necessary, selecting a filler material that can bridge the gap in properties between the base materials is crucial. The choice of filler material should consider factors such as alloy composition, thermal expansion coefficients, and mechanical properties to achieve a weld joint with suitable strength and integrity.
Case Studies
Examples of Successful Welding of Annealed Materials
Case Study 1: Welding of Annealed Carbon Steel Pipes
In this case, annealed carbon steel pipes were successfully welded for a high-pressure fluid transportation system. The welding process involved TIG welding due to its precise control and ability to produce clean welds. Preheating was carefully implemented to avoid excessive thermal gradients and ensure uniform microstructural changes.
The success of the welding process was attributed to:
- Optimal Heat Input: By controlling the heat input during welding, the grain structure of the annealed material was preserved, contributing to the overall weld quality.
- Visual Inspection: Rigorous visual inspection ensured that the welds met the specified criteria for appearance, fusion, and absence of defects.
- Post-Weld Heat Treatment: A controlled post-weld heat treatment was applied to relieve residual stresses and further enhance the mechanical properties of the welded joints.
Challenges Faced in Welding Normalized Materials and Their Solutions
Case Study 2: Welding of Normalized Structural Steel Components
Welding normalized structural steel components for a building project posed challenges related to the prevention of grain growth and maintaining the desired mechanical properties. The components underwent normalization to achieve uniform grain size and improved strength.
Challenges faced and solutions implemented:
- Grain Growth Control: Controlling the welding heat input and employing proper welding techniques, such as low hydrogen processes, minimized the risk of excessive grain growth in the heat-affected zone (HAZ).
- Transformation Behavior: By closely monitoring the transformation behavior during welding, adjustments were made to the preheating temperature and cooling rates, ensuring that the normalized microstructure was retained in the welded joints.
- Post-Weld Quality Checks: Mechanical testing, including bend tests and ultrasonic examinations, were conducted to assess the welds for any defects or deviations from the specified quality criteria.
Strategies for Welding Quenched and Tempered Materials
Case Study 3: Welding of Quenched and Tempered Steel Components
Welding quenched and tempered steel components, such as those used in heavy machinery, presented challenges related to the susceptibility of martensite to cracking and the need to maintain the material’s high strength.
Strategies employed for successful welding:
- Preheating and Controlled Cooling: Preheating the components before welding and carefully controlling the cooling rates helped mitigate the impact of martensitic transformation, reducing the risk of cracking.
- Filler Material Selection: Choosing filler materials with suitable mechanical properties and alloy composition ensured compatibility with the base material, contributing to the overall strength of the welded joints.
- Residual Stress Management: Implementing post-weld heat treatment effectively reduced residual stresses, enhancing the overall stability of the welded components.
FAQs
Is welding annealed materials more challenging than welding normalized materials?
Discussing the challenges and considerations in welding both annealed and normalized materials.
How does the microstructure of materials affect weldability?
Exploring the relationship between microstructure and welding outcomes.
What are the key advancements in welding technology improving weldability?
Highlighting the latest innovations contributing to enhanced weldability.
Can any material be successfully welded, or are there limitations?
Addressing the scope and limitations of welding different materials.
How can welders contribute to environmentally friendly welding practices?
Providing insights into environmentally conscious welding approaches.
Conclusion
In conclusion, recognizing the importance of weldability in material selection and understanding the nuances of welding various materials contribute to the success and safety of engineering projects. The continuous pursuit of knowledge, innovation, and adherence to best practices in welding will shape the future of the industry, ensuring the creation of durable, high-performance structures that stand the test of time.