Welding in Aerospace Fabrication: Crafting the Sky
Introduction
At its core, welding in aerospace fabrication involves the art and science of joining metallic components within the aerospace industry. This process is accomplished by applying heat, pressure, or a combination of both to create a strong, permanent bond between materials. The aerospace sector relies heavily on welding to create intricate and durable components, from the fuselages of aircraft to the critical structures of rocket engines and spacecraft. This field encompasses a wide array of techniques, materials, and stringent quality standards that are paramount in ensuring the safety, reliability, and performance of aerospace vehicles.
Welding in Aerospace Fabrication: A Fusion of Art and Science
In the world of aerospace fabrication, welding serves as the cornerstone, uniting materials and engineering prowess to construct structures that brave the skies. The fusion of artistry and precision engineering empowers aerospace manufacturers to push boundaries and conquer challenges that come with designing aircraft that are lighter, more fuel-efficient, and safer.
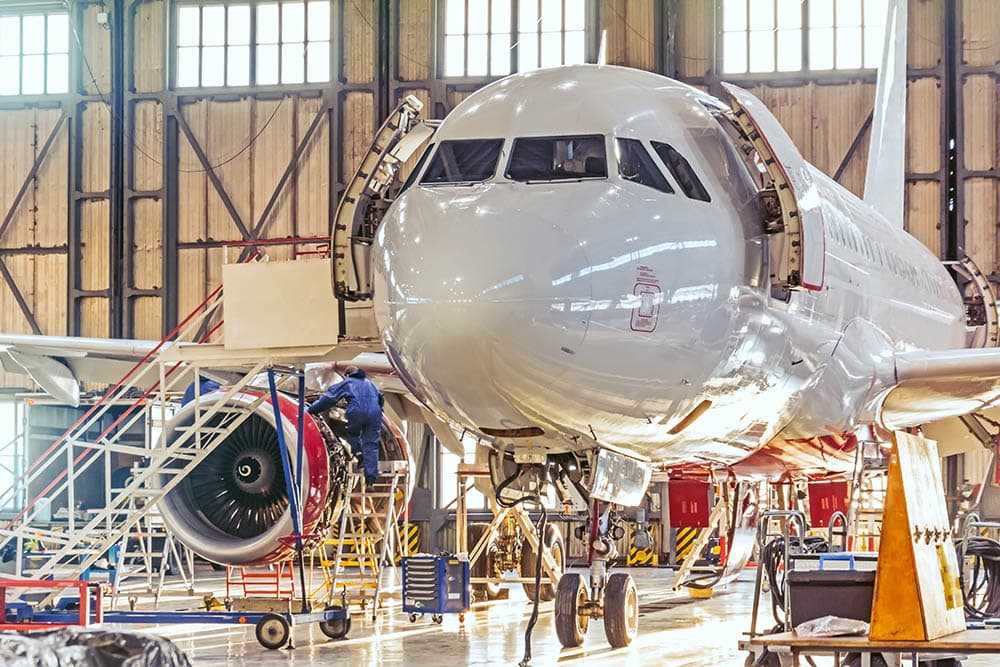
Historical Perspective
Evolution of Welding Techniques in Aerospace Fabrication
The evolution of welding techniques in aerospace fabrication is a captivating journey through time, highlighting the relentless pursuit of perfection in the aerospace industry. From humble beginnings to cutting-edge technology, the methods of joining metals have come a long way.
In the early days of aviation, manual welding processes like oxyacetylene welding were employed. This allowed for the basic joining of materials, but it was far from ideal for the rigorous demands of aerospace. As aviation progressed, arc welding techniques, particularly gas tungsten arc welding (GTAW or TIG) and gas metal arc welding (GMAW or MIG), started to gain prominence. These processes offered greater precision and control, making them suitable for the intricate work required in aerospace fabrication.
However, it was during World War II that welding in aerospace took a giant leap forward. The demand for aircraft production led to significant advancements in welding technology. Electric resistance welding and spot welding, for instance, became vital in the mass production of aircraft components.
The space race of the mid-20th century marked another turning point. The need for lightweight yet strong materials and precision welding techniques led to the development of electron beam welding (EBW) and laser welding. These high-energy processes allowed for the welding of exotic materials like titanium and the construction of spacecraft with unprecedented precision.
Milestones in Aerospace Welding History
The history of aerospace welding is punctuated by several milestones that have left an indelible mark on the industry. Some of the most significant achievements include:
- The Boeing B-29 Superfortress (1940s): This iconic bomber featured extensive use of electric resistance spot welding in its construction, demonstrating the feasibility of mass-producing aircraft using advanced welding methods.
- The Apollo Lunar Module (1960s): Welding played a critical role in the construction of the Apollo Lunar Module. The use of electron beam welding in its fabrication ensured the module’s structural integrity and reliability during the historic moon missions.
- The Space Shuttle Program (1980s): The space shuttle program showcased the application of advanced welding techniques like friction stir welding (FSW). FSW was used to join the large aluminum panels of the shuttle’s external fuel tanks, ensuring both safety and efficiency.
- The Rise of Composite Materials (21st Century): With the introduction of composite materials, aerospace welding techniques had to adapt. Composite-metal hybrid structures required specialized joining methods, leading to innovations in adhesive bonding and composite-metal fusion welding.
Types of Welding Processes in Aerospace Fabrication
Aerospace fabrication relies on a diverse array of welding processes, each tailored to specific materials, joint configurations, and performance requirements. These welding techniques can be broadly categorized into fusion welding, solid-state welding, laser welding, and plasma arc welding.
Fusion Welding: Merging Metal at Its Core
Fusion welding involves the melting of base materials to create a joint. In the aerospace industry, fusion welding techniques are commonly used for their versatility and ability to produce strong, full-penetration welds. Here are some key fusion welding processes:
- Gas Tungsten Arc Welding (GTAW/TIG): GTAW, often referred to as TIG (Tungsten Inert Gas) welding, employs a non-consumable tungsten electrode and an inert shielding gas (such as argon). GTAW is favored for its precision and ability to weld thin materials, making it suitable for aerospace applications where fine control over heat input is crucial.
- Gas Metal Arc Welding (GMAW/MIG): GMAW, also known as MIG (Metal Inert Gas) welding, employs a consumable wire electrode and a shielding gas. It is widely used in aerospace fabrication due to its efficiency and versatility, allowing for both manual and automated welding processes.
- Electron Beam Welding (EBW): EBW is a high-energy welding process that uses a focused beam of electrons to melt and fuse materials together. It is particularly well-suited for aerospace applications involving thick materials or dissimilar alloys. The precision and deep penetration of EBW make it ideal for critical aerospace components.
Solid-State Welding
Solid-state welding techniques do not involve the complete melting of base materials. Instead, they rely on pressure and temperature to create a strong bond between materials. These processes are valued for their ability to preserve material properties and produce high-strength joints:
- Friction Stir Welding (FSW): FSW is a revolutionary solid-state welding technique that employs a rotating, non-consumable tool to mechanically stir and forge materials together at the molecular level. It is commonly used in aerospace for joining aluminum and other lightweight alloys, providing exceptional strength and structural integrity.
- Ultrasonic Welding: Ultrasonic welding utilizes high-frequency vibrations to generate friction and heat at the joint interface, effectively welding materials together. It is often used for joining thermoplastics and some metals in aerospace applications, such as wiring and small components.
- Resistance Welding: Resistance welding encompasses several sub-techniques, including spot welding and seam welding. It relies on the resistance of materials to electric current to generate heat and create welds. Resistance welding is used for various aerospace applications, including sheet metal assembly and electrical connections.
Laser Welding
Laser welding employs a focused laser beam to heat and melt materials, allowing for precise and controlled joining. In aerospace fabrication, laser welding is preferred for its speed and accuracy, making it suitable for both manual and automated processes.
Plasma Arc Welding
Plasma arc welding is a fusion welding process that utilizes a highly focused, electrically conductive plasma arc to melt materials. It offers deep penetration and is often employed in aerospace applications that require a combination of high speed and precision.
Materials and Alloys Used in Aerospace Welding
Aerospace welding requires a deep understanding of materials and alloys to ensure that the final components meet the stringent safety and performance standards of the industry. Here, we explore the common materials and alloys used in aerospace welding, the criteria for selecting them, and the challenges associated with welding aerospace materials.
Common Aerospace Materials
- Aluminum Alloys: Aluminum and its alloys are extensively used in aerospace due to their low density, corrosion resistance, and good strength-to-weight ratio. Common aluminum alloys include 6061, 7075, and 2024, each chosen based on their specific properties for different aerospace components.
- Titanium Alloys: Titanium offers exceptional strength-to-weight ratios, corrosion resistance, and high-temperature stability. Ti-6Al-4V (Grade 5) is a popular choice for aerospace applications, such as aircraft frames and engine components.
- Stainless Steels: Stainless steels are valued for their corrosion resistance, strength, and heat resistance. They find use in aerospace for components like exhaust systems and some structural parts.
- Nickel Alloys: Nickel-based superalloys, like Inconel and Monel, are critical in aerospace for their excellent high-temperature performance, corrosion resistance, and strength. They are used in engine components, exhaust systems, and aerospace turbines.
Material Selection Criteria
The choice of materials for aerospace welding is driven by various factors:
- Weight: Aerospace vehicles aim to minimize weight without compromising strength. Materials with a favorable strength-to-weight ratio, such as aluminum and titanium, are often preferred.
- Strength and Durability: Aerospace materials must withstand extreme forces and environmental conditions, making strength, durability, and resistance to fatigue crucial criteria.
- Corrosion Resistance: Aerospace vehicles are exposed to harsh environments, including high-altitude moisture and salt exposure. Corrosion-resistant materials are essential to ensure long-term performance.
- Temperature Resistance: Components exposed to high temperatures, such as jet engine parts, require materials that can withstand these extremes without deformation or degradation.
- Weldability: The chosen materials must be compatible with the selected welding techniques. Some materials may require special welding processes or filler materials to ensure proper weld quality.
- Cost: While aerospace materials must meet high-performance standards, cost considerations also play a role in material selection, especially for commercial aircraft.
Challenges in Welding Aerospace Materials
Welding aerospace materials presents unique challenges:
- Material Variability: Aerospace materials come in various grades and forms, each with distinct properties. Welding engineers must carefully select the appropriate material for the application.
- Heat-Affected Zone (HAZ): High-temperature welding processes can alter the properties of materials near the weld joint. Minimizing the size and impact of the HAZ is a critical consideration.
- Material Compatibility: Joining dissimilar materials, such as aluminum to titanium, requires precise control of welding parameters and filler materials to prevent material incompatibility issues.
- Residual Stresses: Welding can introduce residual stresses into components, potentially compromising their structural integrity. Post-weld heat treatment and stress-relief techniques are used to mitigate this issue.
- Quality Assurance: Aerospace welding demands rigorous quality control and inspection procedures to detect defects, such as porosity, cracks, and incomplete fusion. Non-destructive testing (NDT) methods like X-ray, ultrasound, and dye penetrant inspection are vital.
Challenges in Aerospace Welding: Striving for Perfection
Welding in aerospace fabrication presents unique challenges that demand innovation and expertise:
Heat-Affected Zone (HAZ): Balancing Strength and Durability
Maintaining material strength while avoiding excessive heat-related distortion is an ongoing battle. Aerospace welders employ advanced cooling techniques and precise heat control to strike this delicate balance.
Material Compatibility: Blending Strengths
Aerospace structures often involve dissimilar materials with varying properties. Achieving strong, reliable joints between these materials is a testament to the artistry of aerospace welding.
Fatigue Resistance: Endurance at Altitude
Aircraft endure immense stress during flight. Welds must exhibit exceptional fatigue resistance to withstand cyclic loading, ensuring the safety and longevity of airborne vehicles.
The Future of Aerospace Welding: Innovations on the Horizon
The landscape of aerospace welding is continuously evolving, driven by technological advancements and industry demands. Key trends include:
Additive Manufacturing: 3D Printing Takes Flight
Additive manufacturing, or 3D printing, is revolutionizing aerospace fabrication. It allows intricate, custom components to be created with reduced material waste, ultimately enhancing efficiency and design possibilities.
Robotic Welding: Precision and Consistency
Robotic welding systems are becoming integral to aerospace fabrication, offering unmatched precision and consistent quality. These automated systems excel at repetitive tasks and complex geometries.
High-Energy Beam Welding: A Fusion of Power
High-energy beam welding methods, like electron and laser beam welding, are evolving to handle thicker materials and larger components. This paves the way for welding larger aerospace structures with increased efficiency.
Welding Standards and Quality Control
Welding standards and quality control are central to the aerospace industry, where precision, reliability, and safety are paramount. This section explores the significance of standards in aerospace welding, key aerospace welding standards, and the rigorous quality control measures implemented to ensure the integrity of aerospace welds.
Importance of Standards in Aerospace Welding
The importance of standards in aerospace welding cannot be overstated. These standards serve as a foundation for achieving consistency, safety, and reliability in the production of aerospace components. Here are several key reasons why standards are crucial:
- Safety: Aerospace vehicles operate in extreme conditions and carry human lives. Welding standards establish guidelines to ensure that welds can withstand these conditions, reducing the risk of catastrophic failure.
- Interchangeability: Aerospace components must fit precisely and function interchangeably. Standards define tolerances and requirements, facilitating the compatibility of parts produced by different manufacturers.
- Performance: Standards set performance criteria for welds, ensuring that they meet the structural and operational requirements of aerospace vehicles. This includes factors like load-bearing capacity, fatigue resistance, and thermal stability.
- Certification and Compliance: Aerospace welding standards are often required for regulatory certification. Compliance with these standards is necessary for obtaining approval from aviation authorities, such as the Federal Aviation Administration (FAA) in the United States.
- Quality Assurance: Standards provide a benchmark for quality control processes. Manufacturers use them to assess the quality of materials, welding procedures, and the final product, ensuring consistency and reliability.
Key Aerospace Welding Standards
Several prominent organizations and governing bodies establish and oversee aerospace welding standards. These standards cover various aspects of welding, including materials, procedures, inspection, and certification. Some key aerospace welding standards include:
- American Welding Society (AWS): The AWS is a significant contributor to welding standards worldwide. In the aerospace sector, standards like AWS D17.1 provide guidelines for the quality assurance and certification of aerospace welding.
- National Aerospace and Defense Contractors Accreditation Program (NADCAP): NADCAP is a global cooperative program that establishes standards for the aerospace and defense industries. It covers processes such as welding, heat treatment, and non-destructive testing.
- European Committee for Standardization (CEN): CEN produces European standards (EN) for aerospace welding, ensuring consistency across European aerospace manufacturers.
- International Organization for Standardization (ISO): ISO standards, such as ISO 3834 for welding quality requirements, are applicable in aerospace manufacturing to guarantee the quality of welded products.
- ASTM International: ASTM standards are widely used for testing and materials specifications in aerospace welding. ASTM E1648, for instance, outlines practices for the radiographic examination of weldments.
Quality Control Measures
Quality control measures are essential in aerospace welding to verify that welds meet the established standards and requirements. These measures include:
- Welder Qualification: Welders must undergo rigorous qualification tests to ensure they possess the necessary skills and expertise. Qualification typically involves welder performance testing (WPT) and visual inspection of welds.
- Welding Procedure Qualification: The welding procedures themselves must be qualified, with detailed documentation of welding parameters, materials, and testing procedures. This ensures that welds are produced consistently to the required standards.
- Non-Destructive Testing (NDT): As discussed earlier, NDT techniques like radiographic testing, ultrasonic testing, and magnetic particle testing are employed to detect defects and ensure the integrity of welds.
- Documentation and Traceability: Detailed records of welding procedures, inspections, and test results are maintained for traceability and audit purposes. This documentation provides a clear history of each weld and component.
- Third-Party Inspections: Independent third-party inspectors may be engaged to verify compliance with standards and quality control processes.
Case Studies
Real-world Examples of Aerospace Welding Applications
Aerospace welding finds application in numerous critical components of aircraft, spacecraft, and satellites. Here are some real-world examples of aerospace welding applications:
- Aircraft Fuselage: Aerospace welding plays a vital role in the construction of aircraft fuselages. Precision welding techniques are used to join aluminum alloy panels, ensuring the structural integrity and airtightness of the fuselage.
- Jet Engine Components: In jet engines, nickel-based superalloys like Inconel are welded to form components such as turbine blades and exhaust systems. Electron beam welding and laser welding are often used for these high-temperature applications.
- Rocket Propulsion: Rocket engines demand robust welds to withstand extreme temperatures and pressures. Friction stir welding is employed to join the components of rocket engine nozzles and chambers.
- Spacecraft Structures: Welding is utilized in the construction of spacecraft structures, where lightweight materials like titanium and aluminum are welded to create frames, supports, and payload bays.
- Satellite Components: Satellite manufacturers rely on precision welding for critical components such as antennas, solar panel frames, and propulsion systems.
Success Stories and Lessons Learned
- SpaceX and the Falcon 9: SpaceX, under the leadership of Elon Musk, has revolutionized aerospace with its reusable Falcon 9 rocket. The company’s innovative approach to welding and materials, along with the use of advanced aluminum-lithium alloys, has significantly reduced launch costs and expanded access to space.
- Boeing 787 Dreamliner: The Boeing 787 Dreamliner features extensive use of composite materials and advanced welding techniques. Its success demonstrates the benefits of materials innovation and precision welding in the aerospace industry.
- Hubble Space Telescope Repair: The Hubble Space Telescope’s initial deployment revealed a flaw in its primary mirror, affecting its ability to capture clear images. A successful repair mission in 1993, which included the installation of corrective optics, showcased the importance of precision welding in space operations.
- Mars Rover Perseverance: The Mars Rover Perseverance, a flagship mission by NASA, utilized advanced welding techniques to assemble its complex robotic arm and instrumentation. The rover’s successful landing and ongoing mission exemplify the critical role of welding in planetary exploration.
- Lessons Learned: Aerospace welding has evolved significantly, with lessons learned from past failures and successes. Failures like the Space Shuttle Challenger disaster in 1986 underscored the importance of rigorous quality control and adherence to welding standards in the aerospace industry. Conversely, the successful Apollo missions to the moon demonstrated the resilience and reliability of aerospace welds in extreme conditions.
Notable Aerospace Welding Projects
- International Space Station (ISS): The ISS is a remarkable example of international collaboration and advanced welding technology. Precision welding played a critical role in constructing this space laboratory, where multiple nations’ contributions were seamlessly integrated.
- Orion Spacecraft: NASA’s Orion spacecraft, designed for future deep-space exploration, relies on advanced welding techniques for its pressurized crew module. The spacecraft’s missions, including planned missions to the Moon and Mars, highlight the significance of robust welding in long-duration spaceflight.
- Space Launch System (SLS): NASA’s Space Launch System, intended for deep-space missions, features large welded structures, including the core stage and solid rocket boosters. Welding innovations have been instrumental in the development of this powerful launch vehicle.
- Commercial Spacecraft: Private companies like Blue Origin and Virgin Galactic are pioneering commercial space travel. Welding is central to their spacecraft, contributing to the growth of commercial space ventures and space tourism.
FAQs
Can traditional welding techniques meet the demands of modern aerospace fabrication?
Traditional techniques still play a vital role, but advancements like laser and electron beam welding cater to intricate aerospace designs and materials.
How does additive manufacturing impact aerospace welding?
Additive manufacturing complements traditional welding by enabling the creation of complex components with minimal material waste, streamlining the production process.
What role does human expertise play in robotic welding?
While robotic welding systems offer precision, human expertise remains critical for programming, quality control, and adapting to unique challenges.
How do aerospace welders ensure fatigue resistance?
Welders employ techniques like shot peening and post-weld heat treatment to enhance the fatigue resistance of welds, prolonging the life of aerospace structures.
What’s the significance of high-energy beam welding in aerospace?
High-energy beam welding methods are crucial for joining thick materials, ensuring structural integrity in larger aerospace components.
How does aerospace welding contribute to aircraft safety?
Aerospace welding directly impacts aircraft structural integrity, ensuring that every joint and weld can withstand the stresses of flight, enhancing passenger safety.
Conclusion: Forging Dreams, One Weld at a Time
In the realm of aerospace fabrication, welding stands as a testament to human ingenuity and perseverance. As we journey through the skies on magnificent flying machines, let’s not forget the skilled welders and engineers who craft these dreams into reality, soaring beyond the boundaries of possibility.