Post Weld Heat Treatment Process (PHWT)
In the field of welding, achieving strong and reliable joints is crucial for ensuring the structural integrity and performance of various components. One effective method employed to enhance the properties of weldments is the Post Weld Heat Treatment Process (PHWT). This process involves subjecting welded materials to carefully controlled heating and cooling cycles, which significantly improve their mechanical properties.
Post Weld Heat Treatment Process (PHWT)
The Post Weld Heat Treatment Process (PHWT), also known as post-weld heat treatment or PWHT, is a thermal treatment applied to welded materials to refine their microstructure and relieve residual stresses. By subjecting the welded components to controlled heating and subsequent cooling, the PHWT process enhances the mechanical properties, such as strength, toughness, and ductility, while reducing the risk of cracking and distortion.
The PHWT process typically involves heating the welded structure to a specific temperature range, holding it at that temperature for a predetermined period, and then gradually cooling it down. The selection of the temperature range and duration depends on factors such as the material composition, welding process used, and desired properties. Proper control and adherence to specific heat treatment parameters are critical to achieving the desired results.
Types of Post Weld Heat Treatment
Stress-relieving PWHT
- Definition and purpose: Stress-relieving post weld heat treatment (PWHT) is a heat treatment process performed on welded components to reduce residual stresses and minimize distortion that may have been introduced during the welding process. The primary purpose of stress-relieving PWHT is to improve the mechanical integrity and dimensional stability of the welded structure.
- Effects on residual stresses and distortion:Welding processes generate localized heating and cooling, causing significant thermal gradients within the welded structure. These temperature differentials result in the formation of residual stresses that can affect the mechanical properties and long-term performance of the weld. Stress-relieving PWHT helps to redistribute these residual stresses by subjecting the welded component to a carefully controlled heating and cooling cycle, reducing the stress concentrations. The heat treatment process also helps in minimizing distortion. Distortion in welded structures can occur due to thermal expansion and contraction during welding. Stress-relieving PWHT helps alleviate these residual stresses, reducing the risk of distortion and ensuring dimensional stability.
- Thermal Stress Relief: This method involves heating the welded component to a specific temperature, typically below the lower transformation temperature, and maintaining it for a predetermined duration. The component is then gradually cooled. The heating and cooling rates are controlled to prevent the introduction of additional thermal stresses.
- Vibratory Stress Relief: Vibratory stress relief utilizes mechanical vibrations applied to the welded structure to induce micro-plastic deformation, which helps in reducing residual stresses. This method is particularly suitable for large structures or components where traditional heat treatment may not be feasible.
- High-Frequency Stress Relief: High-frequency stress relief utilizes high-frequency electromagnetic fields to induce resistive heating in the welded component, resulting in stress relief. This method is commonly used for localized stress relief in specific areas of the weld.
- Temperature and duration consideration: The temperature and duration of stress-relieving PWHT are critical factors that determine its effectiveness. The temperature must be carefully selected based on the material composition, thickness, and welding procedure used. The typical temperature range for stress relief is between 500°C and 700°C (932°F and 1292°F). The duration of the treatment depends on the size and complexity of the welded structure but generally ranges from a few hours to several days.It is essential to ensure that the selected temperature does not exceed the material’s upper transformation temperature to avoid unintended phase changes or degradation of material properties. Similarly, the heating and cooling rates should be controlled to minimize the risk of thermal shock or the introduction of additional stresses. The specific temperature and duration requirements for stress-relieving PWHT are typically provided by industry standards, codes, or project specifications, taking into account the material type, welding procedure, and structural requirements.
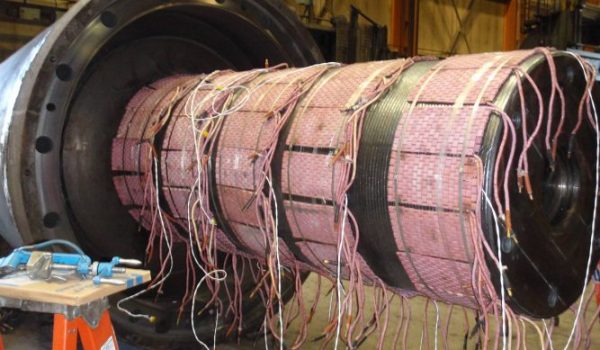
Preheat and Interpass PWHT
Preheat and interpass PWHT are heat treatment processes performed before and during the welding process, respectively. The purpose of preheat and interpass PWHT is to control the temperature of the base metal and weld joint during welding, thereby influencing the material properties, microstructure, and overall weld integrity.
Effects on material properties and Microstructure
- Reduction of hydrogen-induced cracking: Preheating the base metal before welding helps in reducing the risk of hydrogen-induced cracking. By raising the base metal temperature, preheating allows hydrogen to diffuse out more easily, minimizing its detrimental effects on the weld joint’s integrity.
- Improved weldability: Preheating also improves the weldability of certain materials by reducing the cooling rate and thermal gradients during welding. This helps to prevent the formation of brittle phases and reduces the likelihood of cracking.
- Grain refinement: Preheating and interpass PWHT can promote grain refinement in the heat-affected zone (HAZ) and the weld metal. Controlled heating and cooling rates influence the microstructural evolution, resulting in smaller and more uniform grains. This can enhance the mechanical properties, such as toughness and fatigue resistance, of the weld joint.
- Stress reduction: Preheating and interpass PWHT can help mitigate residual stresses introduced during welding. By controlling the temperature gradients, these processes minimize the formation of high residual stresses, leading to improved weld integrity.
Preheat and interpass temperature guidelines
The selection of preheat and interpass temperatures depends on various factors, including the material type, thickness, welding procedure, and applicable codes or standards. The specific guidelines may vary for different materials and applications. Here are some general considerations:
- Material thickness: Thicker materials generally require higher preheat temperatures to reduce the cooling rate and prevent excessive thermal stress. The thicker the material, the longer the heat needs to be applied before welding.
- Material type: Different materials have varying susceptibility to cracking and microstructural changes. Guidelines for preheat and interpass temperatures are typically provided based on the material’s weldability and specific requirements.
- Welding procedure: The welding procedure, including the welding process, filler material, and joint design, can influence the preheat and interpass temperature requirements. The procedure should be optimized to achieve a balance between weldability, microstructure, and desired mechanical properties.
Influence on weld integrity and joint strength
Preheat and interpass PWHT significantly influence weld integrity and joint strength by controlling material properties and reducing the risk of defects. The key effects include:
- Reduction of cracking: Preheating and interpass PWHT minimize the risk of hydrogen-induced cracking, solidification cracking, and other types of weld defects. This ensures the structural integrity of the weld joint.
- Improved toughness: Proper temperature control during preheat and interpass PWHT promotes the formation of fine-grained microstructures, enhancing the toughness and resistance to brittle fracture of the weld joint.
- Enhanced joint strength: By reducing residual stresses and optimizing microstructural properties, preheat and interpass PWHT contribute to improved joint strength and overall weld quality.
Hardening PWHT
Definition and purpose
Hardening PWHT, also known as post weld hardening, is a heat treatment process applied to certain materials and weldments to increase their hardness and improve wear resistance. The purpose of hardening PWHT is to enhance the mechanical properties of the weld joint, particularly its hardness, without compromising other essential characteristics.
Induction and flame hardening techniques
- Induction hardening: Induction hardening involves heating the weld joint using high-frequency electromagnetic fields. The localized heating raises the temperature of the weld metal and adjacent base metal, followed by rapid quenching to achieve the desired hardness. Induction hardening is commonly used for small to medium-sized components with complex shapes.
- Flame hardening: Flame hardening is a process where the weld joint is heated using an oxy-fuel flame. The heat source is directed onto the desired area, and the heated zone is then rapidly cooled using water or other quenching media. Flame hardening is suitable for larger components or specific areas requiring localized hardening.
Materials suitable for hardening PWHT:
Hardening PWHT is typically applied to carbon steels, alloy steels, and certain stainless steels. The specific material selection depends on the desired hardness, wear resistance requirements, and compatibility with the welding process. Some commonly hardened materials include:
- Low carbon steels: Low carbon steels can be effectively hardened through PWHT to improve their hardness and wear resistance, making them suitable for applications involving abrasive wear or impact loading.
- Medium to high carbon steels: Medium to high carbon steels can undergo hardening PWHT to achieve even higher hardness levels and improved strength. These materials are commonly used in applications requiring high wear resistance, such as gears, shafts, and tools.
- Alloy steels: Alloy steels with specific alloying elements, such as chromium, molybdenum, or nickel, can benefit from hardening PWHT. The process enhances their hardness, strength, and wear resistance, making them suitable for demanding applications in industries such as automotive, aerospace, and tooling.
- Certain stainless steels: Some stainless steels can be subjected to hardening PWHT to increase their hardness and wear resistance. However, it is important to note that not all stainless steels are suitable for this process, as some may experience undesirable changes in corrosion resistance or other properties.
PWHT Process Parameters
Temperature control and monitoring
- Temperature control: Precise temperature control is essential in PWHT to ensure the desired heat treatment effects. The temperature should be accurately maintained within the specified range throughout the heating and holding periods. Advanced temperature control systems, such as thermocouples or infrared pyrometers, are commonly used to monitor and regulate the temperature.
- Temperature uniformity: Uniform temperature distribution is important to achieve consistent heat treatment results. Proper positioning of heating elements, thermal insulation, and circulation of heat within the furnace or heat treatment chamber help ensure uniform temperature across the welded component.
- Temperature monitoring: Continuous monitoring of the temperature during PWHT is necessary to detect any deviations or fluctuations. Temperature data loggers or control systems provide real-time temperature readings, allowing operators to make necessary adjustments to maintain the desired temperature profile.
Heating and cooling rates
- Heating rates: The rate at which the welded component is heated during PWHT should be controlled to avoid thermal shock, distortion, or the introduction of additional stresses. Slow and controlled heating rates are generally preferred to minimize temperature gradients and allow for uniform expansion.
- Cooling rates: The cooling rate is crucial in achieving the desired microstructure and properties. Depending on the material and specific requirements, different cooling rates may be employed. Some PWHT processes require controlled cooling rates, while others involve rapid quenching to achieve specific hardening effects.
Holding time and soak periods
- Holding time: The holding time refers to the duration that the welded component is maintained at the specified PWHT temperature. It allows for the desired transformation or diffusion processes to occur. Holding times can vary depending on the material, size, and specific heat treatment requirements. It is typically determined based on industry standards, codes, or project specifications.
- Soak periods: Soak periods are specific time intervals during PWHT when the component is held at a constant temperature for a sufficient period to allow for thermal equilibrium and transformation. Soak periods are important for achieving uniform microstructural changes throughout the weldment.
Material-specific considerations
- Material composition: Different materials exhibit varying response to PWHT. Material-specific considerations include the phase transformation temperatures, hardenability, susceptibility to cracking, and other material properties. The selection of PWHT parameters should be based on a thorough understanding of the material characteristics.
- Material thickness: The thickness of the welded component influences the PWHT process parameters. Thicker materials require longer heating and holding times to ensure adequate heat transfer and achieve the desired effects throughout the entire cross-section.
Equipment and tools used in PWHT
- Furnaces or heat treatment chambers: Furnaces or heat treatment chambers are commonly used for PWHT. They provide a controlled environment for heating, temperature maintenance, and cooling. Different types of furnaces, such as electric resistance furnaces or gas-fired furnaces, may be used depending on the specific requirements.
- Thermocouples and temperature sensors: Thermocouples and temperature sensors are essential for accurate temperature measurement and monitoring during PWHT. They are typically placed at strategic locations on the welded component to ensure proper temperature control and uniformity.
- Quenching equipment: For processes involving rapid cooling or quenching, specialized equipment such as water or oil quench tanks, quenching jets, or spray systems may be used to achieve the desired cooling rate and hardness.
- Data loggers and control systems: Data loggers and control systems are employed to record and monitor temperature profiles, heating and cooling rates, and other process parameters. They provide real-time data for process control and documentation.
Factors Influencing PWHT
Material composition and properties
- Material response to heat treatment: Different materials have varying responses to PWHT due to their composition and properties. Factors such as phase transformation temperatures, hardenability, susceptibility to cracking, and thermal conductivity influence the selection of PWHT parameters. Materials with higher hardenability may require more stringent heat treatment conditions to achieve the desired properties.
- Material strength and toughness: The initial strength and toughness of the base metal and weld joint influence the PWHT requirements. Weaker or more brittle materials may require specific temperature and cooling rate profiles to avoid excessive softening or increased brittleness during heat treatment.
- Material sensitivities: Some materials may be sensitive to certain heat treatment conditions, such as rapid heating or cooling rates, leading to the risk of distortion, cracking, or undesirable changes in microstructure. It is important to consider any material sensitivities when determining the PWHT parameters.
Welding method and process
- Welding heat input: The heat input during welding affects the extent of thermal cycles experienced by the weld joint. Higher heat inputs can lead to larger heat-affected zones and higher residual stresses. The PWHT process parameters may be adjusted accordingly to account for the specific welding method and heat input.
- Filler material selection: The choice of filler material can impact the PWHT requirements. Some filler materials may have different transformation behavior or alloying elements that affect the heat treatment response. The compatibility between the base metal, filler material, and PWHT should be considered.
- Weld joint design: The geometry and configuration of the weld joint can influence the PWHT process. Thick or complex weld joints may require longer heating and holding times to ensure proper heat distribution and achieve the desired effects throughout the entire joint.
Thickness and geometry of welded components
- Component thickness: The thickness of the welded component affects the heat transfer and thermal gradients during PWHT. Thicker materials require longer heating and holding times to ensure adequate heat penetration and uniform transformation.
- Component geometry: Complex or intricate component geometries can present challenges in achieving uniform temperature distribution during PWHT. Considerations such as the placement of thermocouples, heating methods, and insulation techniques should be taken into account to ensure consistent heat treatment throughout the entire weldment.
Post-weld inspection and testing requirements
- Code and standard compliance: Certain codes and standards may specify specific PWHT requirements based on the intended application or industry. Adherence to these requirements is essential to ensure compliance and the desired level of quality and integrity.
- Non-destructive testing (NDT): Post-weld inspection and testing methods, such as radiographic testing (RT), ultrasonic testing (UT), or magnetic particle inspection (MPI), may be required to assess the weld joint’s quality and detect any defects. The PWHT parameters may need to be adjusted to ensure that any potential defects are adequately addressed during the heat treatment process.
- Mechanical testing: Mechanical testing, such as tensile testing or impact testing, may be performed to evaluate the mechanical properties of the weld joint after PWHT. The PWHT parameters should be selected to achieve the desired mechanical properties specified by the applicable requirements.
Benefits of Post Weld Heat Treatment Process (PHWT)
The application of the Post Weld Heat Treatment Process (PHWT) offers several benefits that contribute to the overall quality and reliability of welded structures. Some of the key advantages include:
- Improved Mechanical Properties: The PHWT process refines the microstructure of welded materials, leading to improved mechanical properties. This includes increased strength, toughness, and ductility, making the weldments more resistant to deformation and failure under load.
- Residual Stress Relief: Welding induces residual stresses in the welded components due to non-uniform heating and cooling. The PHWT process helps alleviate these stresses, reducing the risk of stress corrosion cracking and distortion. By relieving residual stresses, the post-weld heat treatment enhances the structural integrity and dimensional stability of the weldments.
- Mitigation of Hydrogen Embrittlement: Certain welding processes, such as shielded metal arc welding (SMAW) and gas metal arc welding (GMAW), can introduce hydrogen into the weld metal. Hydrogen can cause embrittlement, leading to catastrophic failure under stress. Post weld heat treatment helps mitigate hydrogen embrittlement by promoting the release of trapped hydrogen, ensuring the integrity of the welded joint.
- Refinement of Weld Zone Microstructure: The PHWT process promotes the formation of a refined and uniform microstructure in the weld zone. This results in improved grain structure, reduced segregation of alloying elements, and elimination of defects such as inclusions and porosity. The refined microstructure enhances the overall quality and performance of the welded joint.
- Controlled Heat-Affected Zone (HAZ) Properties: The heat-affected zone (HAZ) adjacent to the weld can undergo changes in its microstructure and properties during welding. Post weld heat treatment helps control and optimize the properties of the HAZ, ensuring that it possesses desirable characteristics and avoids potential issues such as brittle fracture.
Applications of Post Weld Heat Treatment Process (PHWT)
The Post Weld Heat Treatment Process (PHWT) finds extensive applications across various industries where welded components are employed. Some notable applications of PHWT include:
- Oil and Gas Industry: In the oil and gas industry, welded components such as pipelines, pressure vessels, and storage tanks are subjected to harsh operating conditions. Post weld heat treatment is commonly employed to ensure the integrity and reliability of these critical structures. PHWT helps improve the resistance to corrosion, fatigue, and brittle fracture, ensuring the long-term performance of weldments in oil and gas facilities.
- Power Generation: Welded components in power generation plants, such as boilers, turbines, and piping systems, are exposed to high temperatures and pressures. The PHWT process plays a vital role in optimizing the mechanical properties of these weldments, ensuring their ability to withstand demanding operational conditions and reducing the risk of failures.
- Aerospace and Automotive Industries: Welded structures in the aerospace and automotive industries undergo stringent quality and safety requirements. Post weld heat treatment is often employed to enhance the strength and fatigue resistance of critical components such as aircraft frames, engine mounts, and automotive chassis, ensuring their structural integrity under extreme loads and conditions.
- Heavy Machinery and Equipment: Welded structures in heavy machinery, such as construction equipment, mining machinery, and cranes, are subjected to heavy loads and intense vibrations. The PHWT process helps optimize the mechanical properties of these weldments, improving their load-bearing capacity, fatigue life, and resistance to cracking and failure.
- Pressure Vessels and Chemical Process Equipment: Pressure vessels and chemical process equipment, including reactors, columns, and heat exchangers, require reliable weldments to ensure safe operation. Post weld heat treatment is commonly applied to these components to enhance their structural integrity, corrosion resistance, and resistance to stress corrosion cracking.
- Shipbuilding and Offshore Structures: Welded structures in the shipbuilding and offshore industries face challenging environmental conditions, including corrosive seawater and dynamic loads. The PHWT process is essential to improve the weldment properties, such as toughness, corrosion resistance, and fatigue strength, ensuring the durability and safety of marine and offshore structures.
FAQs
What is the purpose of Post Weld Heat Treatment Process (PHWT)?
The purpose of the Post Weld Heat Treatment Process (PHWT) is to refine the microstructure of welded materials, relieve residual stresses, and enhance the mechanical properties of weldments, improving their strength, toughness, and ductility.
When is Post Weld Heat Treatment necessary?
Post weld heat treatment is necessary in cases where the welded structures require improved mechanical properties, such as strength, toughness, or resistance to corrosion and fatigue. It is particularly crucial for critical applications in industries like oil and gas, power generation, and aerospace.
Which welding processes can benefit from Post Weld Heat Treatment?
Various welding processes can benefit from post weld heat treatment, including shielded metal arc welding (SMAW), gas metal arc welding (GMAW), flux-cored arc welding (FCAW), submerged arc welding (SAW), and more. The specific process and material used determine the suitability and parameters of the heat treatment.
What are the temperature ranges typically used in Post Weld Heat Treatment?
The temperature ranges used in post weld heat treatment depend on the material being welded. Common temperature ranges include 500-800°C (932-1472°F) for low-carbon steels and 600-1100°C (1112-2012°F) for high-strength alloy steels.
How long does Post Weld Heat Treatment take?
The duration of post weld heat treatment varies based on factors such as material type, thickness, and desired properties. It can range from a few hours to several days. Proper heating and cooling rates, as well as holding times, are crucial to achieving the desired results.
How does Post Weld Heat Treatment relieve residual stresses?
Post weld heat treatment relieves residual stresses by subjecting the welded structure to controlled heating and cooling cycles. The thermal expansion and contraction during heating and cooling help redistribute and alleviate the internal stresses, reducing the risk of stress corrosion cracking and distortion.
Can all welded components undergo Post Weld Heat Treatment?
Not all welded components can undergo post weld heat treatment. Some factors, such as material composition, size, and shape, may restrict or limit the feasibility of heat treatment. It is essential to consult with materials and welding engineers to determine the applicability of post weld heat treatment for specific weldments.
Conclusion
The Post Weld Heat Treatment Process (PHWT) plays a significant role in enhancing the structural integrity and performance of welded components in various industries. By subjecting welded structures to controlled heating and cooling cycles, the PHWT process refines the microstructure, relieves residual stresses, and improves mechanical properties such as strength, toughness, and ductility. The benefits of post weld heat treatment extend to critical applications in oil and gas, power generation, aerospace, and many other sectors. With proper control and adherence to specific heat treatment parameters, the PHWT process ensures the long-term reliability and safety of welded structures. Embracing post weld heat treatment as an integral part of the welding process can lead to improved quality, durability, and performance of welded components, ultimately benefiting industries and end-users alike.